The Role of Satellites in 5G Networks
NOTE: This is an excerpt from a policy brief on the data-centric national security risks associated with reliance on satellite segments in 5G telecommunications networks. For a more in-depth examination of the role of satellites in 5G networks read, “Seizing Opportunities." To learn more about 5G networks more broadly, visit 5G.wilsoncenter.org.
For the promise of the fifth generation of mobile networks (5G) to be fully realized (i.e. nearly ubiquitous, instantaneous, connectivity for large numbers of devices globally), terrestrial telecommunications systems, which heavily rely on buried fiber optic cables, will not be enough.
Instead, we will need to move from (a) largely separate satellite and terrestrial communications systems with satellites used primarily for solving “the last mile” problem (areas where laying fiber was a physical or economic challenge) or for discrete use cases (e.g. processing credit card payments at gas station) to (b) an integrated 5G ‘network of networks’ where satellites play an increasing role alongside terrestrial networks.
Why? While the utility of satellite communications is more limited within cities and in city-to-city communications (areas where fiber and WIFI already dominate and the lines of sight necessary for satellites are significantly reduced), integrating satellite and terrestrial systems will be necessary to meet the full spectrum of future demands likely to be placed on 5G networks. These include:
- increasing traffic and number of connections outside of dense city centers in more rural and remote areas with the proliferation of Internet of Things (IoT) devices,
- providing coverage for devices on the move (such as a ship at sea or a car driving across the United States), and
- processing and data caching pushing progressively closer and closer to the networks’ edge (i.e. edge computing) and farther away from areas of dense fiber availability.
Take, for example, the connectivity needs of mobility. If you disconnect a mobile asset (car, truck, plane, drone, ship) from the fiber network, you can still keep it connected by WIFI and terrestrial 5G infrastructure so long as it is in or is in close proximity to cities. But as you move to more rural and remote areas, only satellite communication (SATCOM) has the potential to provide reliable coverage and sufficient data density. As the number, uses, and requirements of connectivity continue to evolve, so does the importance of extending the promise of 5G networks beyond the urban and densely networked communities.
To meet these demands, satellites will need to serve a diversity of purposes ranging from the “last mile” problem to connections on the move, redundancy for critical emergency services, edge networking, and IoT dense traffic areas outside of the already highly networked cities.
In short, satellites will play a key role in determining our collective 5G future. How we integrate terrestrial and space-based components will determine the type and degree of connectivity 5G networks enable in practice across the United States and around the world, rather than what they could have enabled in theory.
As the number, uses, and requirements of connectivity continue to evolve, so does the importance of extending the promise of 5G networks beyond the urban and densely networked communities.
As a consequence, 5G represents more than just an important shift in (a) the possibilities for, (b) functions of, and (c) the hardware-software interplay in mobile telecommunications networks as we moved from legacy generations such as 3G and more modern generations like 4G LTE and now to 5G. 5G also represents an opportunity for a shift in the relationship between terrestrial and space-based communications systems more broadly.
And, importantly, for the first time, an evolution of the underpinning technology (primarily satellite and launch technology) and the business models of satellite companies (including satellites as a service) makes this integration both technically possible and economically feasible.
This shift can be seen today by the emergence of companies like SpaceX, OneWeb, AST SpaceMobile, and Project Kupier, who seek to create disruptive business models in low earth orbit (LEO), as well as more traditional geosynchronous (GSO) providers like Telesat and ViaSat who are adapting their infrastructure and satellite capabilities to compete for market share with the proliferated LEO providers.
Putting Orbits in Context
-
Low Earth Orbit (LEO)
-
Medium Earth Orbit (MEO)
-
Geosynchronous Orbit (GSO)
-
Geostationary Orbit (GEO)
This convergence between terrestrial and space-based telecommunications networks for 5G is not merely hypothetical. Earlier this year (2021), Lockheed Martin’s space division announced a strategic partnership with satellite start-up Omnispace to jointly build out a space-based 5G network. Their hybrid network seeks to combine satellite and mobile wireless carrier networks to create “a global 5G network, which enables users to seamlessly transition between the satellite [and the] terrestrial network.” Notably, this hybrid network would be the first integrated 5G platform for commercial and government use featuring space-based and terrestrial network architectures.
Taken together, these three factors - (i) evolving satellite system technology and (ii) business models coupled with (iii) growing demand for bandwidth - make it possible (though not inevitable) for satellites to have an increasing role in telecommunications networks in general and 5G networks in particular. However, the potential for this evolving role is still largely overlooked and/or poorly or largely misunderstood. Moreover, while 5G is best understood as a network of networks, which can include satellites, many have overlooked or are unaware of that fact. Much of our focus on the national security implications of 5G to date has been on the terrestrial components of these networks: Internet of Things (IoT) and mobile devices, radio heads and towers, fiber, the core network, etc.
When we say “satellites”, what do we mean?
‘Satellites’ is often used as shorthand for satellite systems. Satellite systems consist of four basic segments: ground-based assets, space-based assets, links between elements (i.e. uplinks, downlinks, and crosslinks), and connection points to other networks or devices (the users or customers of these systems).
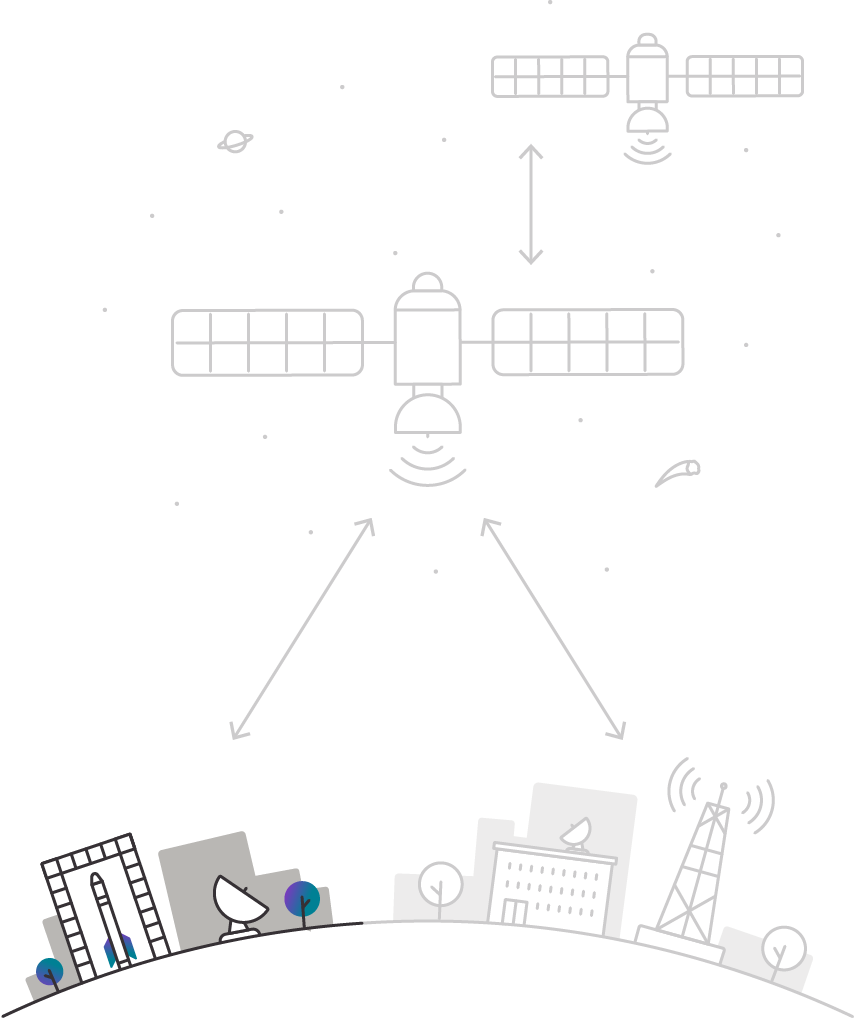
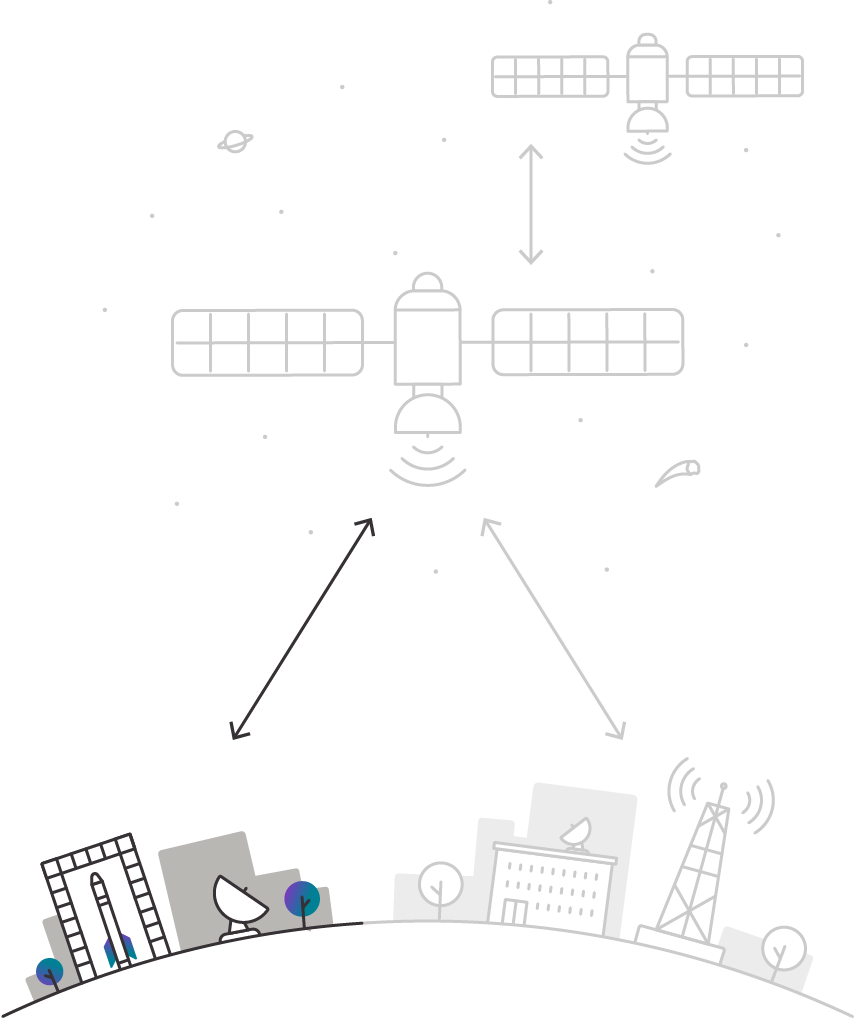
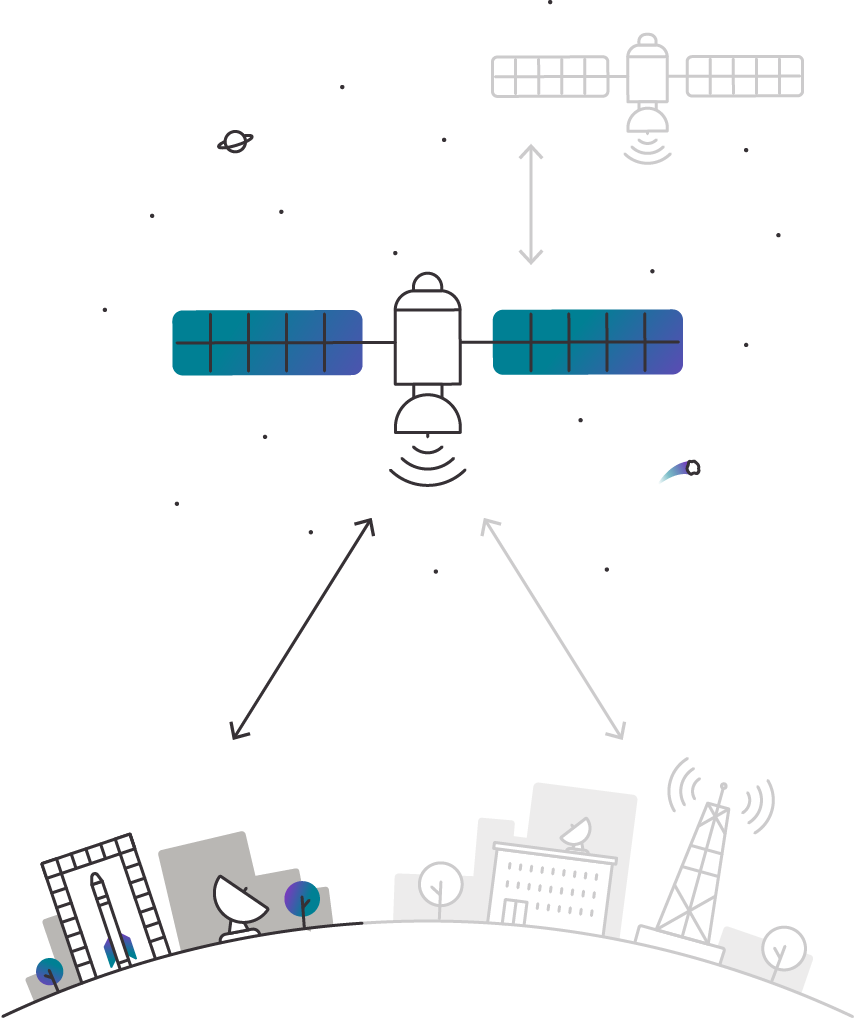
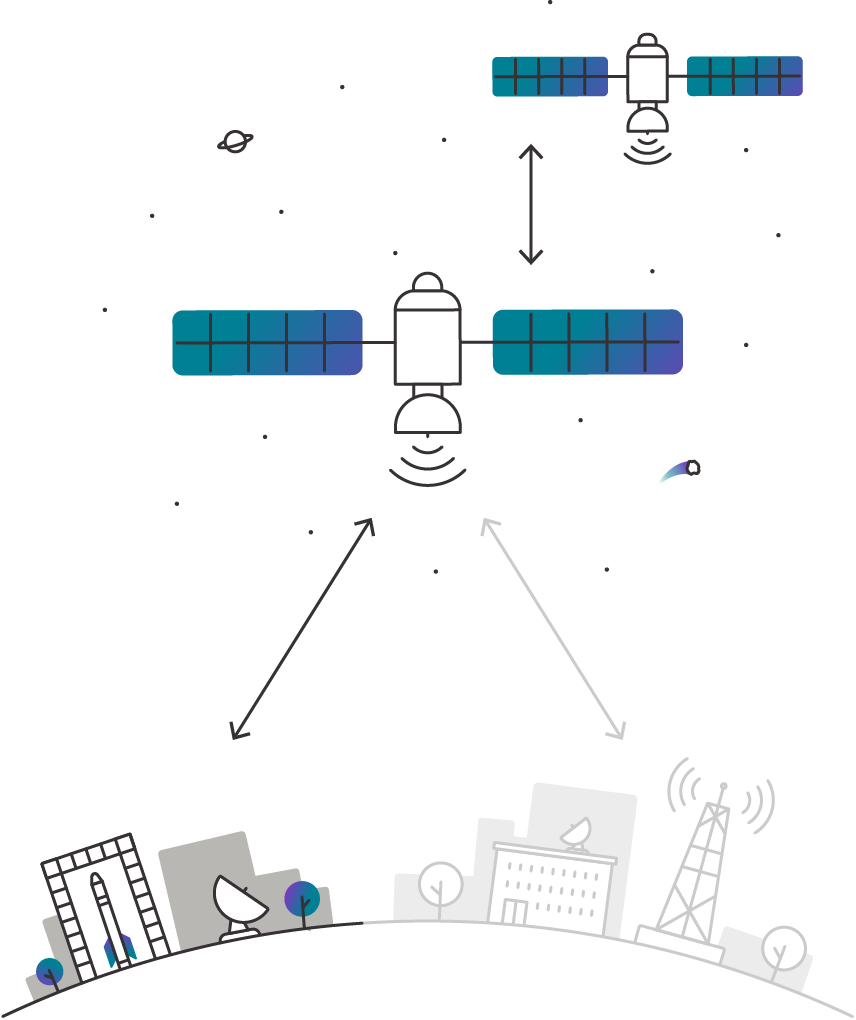
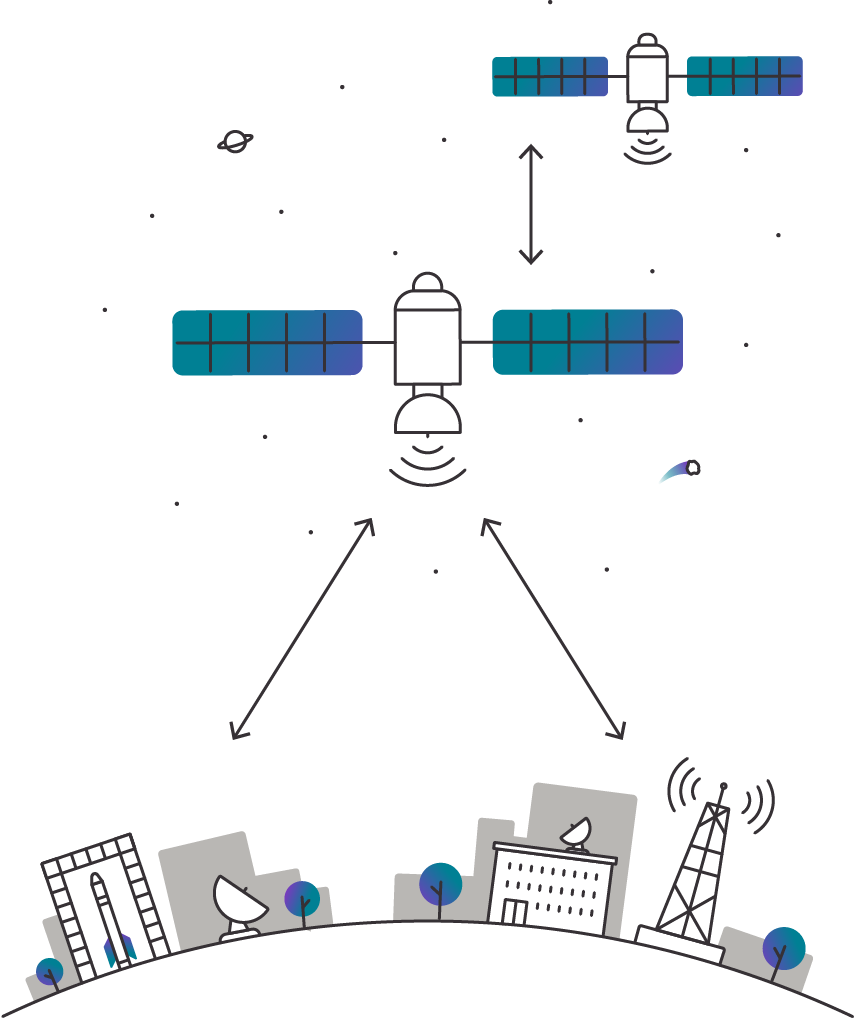
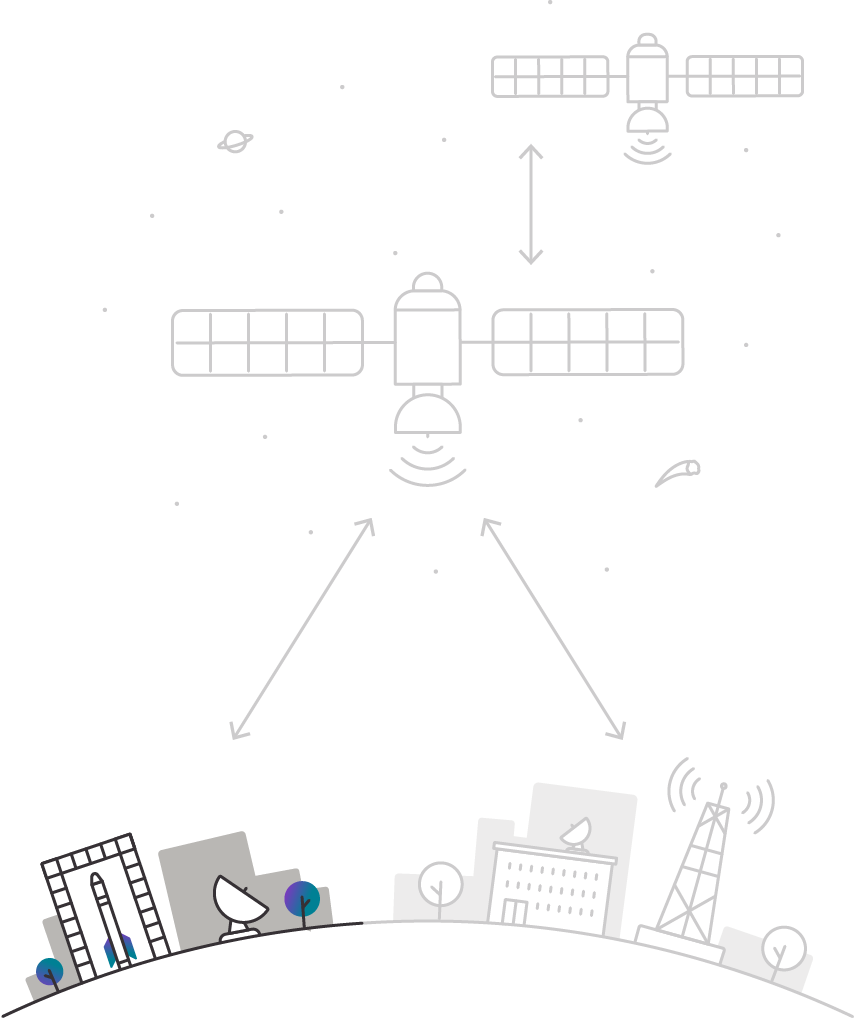
Ground-based Assets
Ground-based assets are terrestrial (surface-based facilities) and include ground stations and launch facilities. Launch facilities make it possible to put a satellite into space and are historically operated by a company that is distinct from those operating the satellites in practice. Ground stations are the brain of the entire satellite networks. They serve as control systems for the satellites in orbit and, as a consequence, if something goes wrong with a satellite in space, the personnel at these stations will be the first to know and in the best position to carry out incident response.
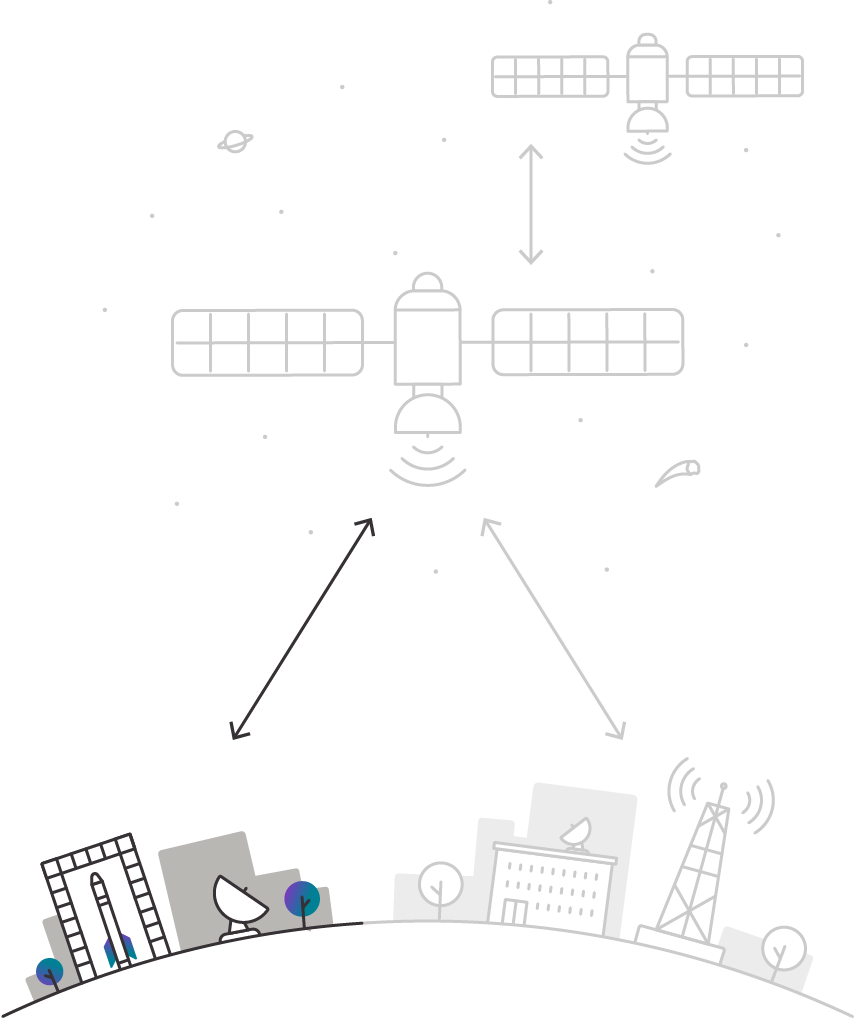
Uplinks & Downlinks
These stations provide real-time communications with satellites enabling telemetry, tracking, and control (TT&C) over satellite networks as well as managing uplinks (sending radio signals to the satellite) and downlinks (receiving data transmission from the satellite). When people hear satellite systems, it is often the satellite that stands out and tends to garner the most attention. However, these satellites are only effective if they have somewhere to send and act on their data (i.e. ground-based assets).
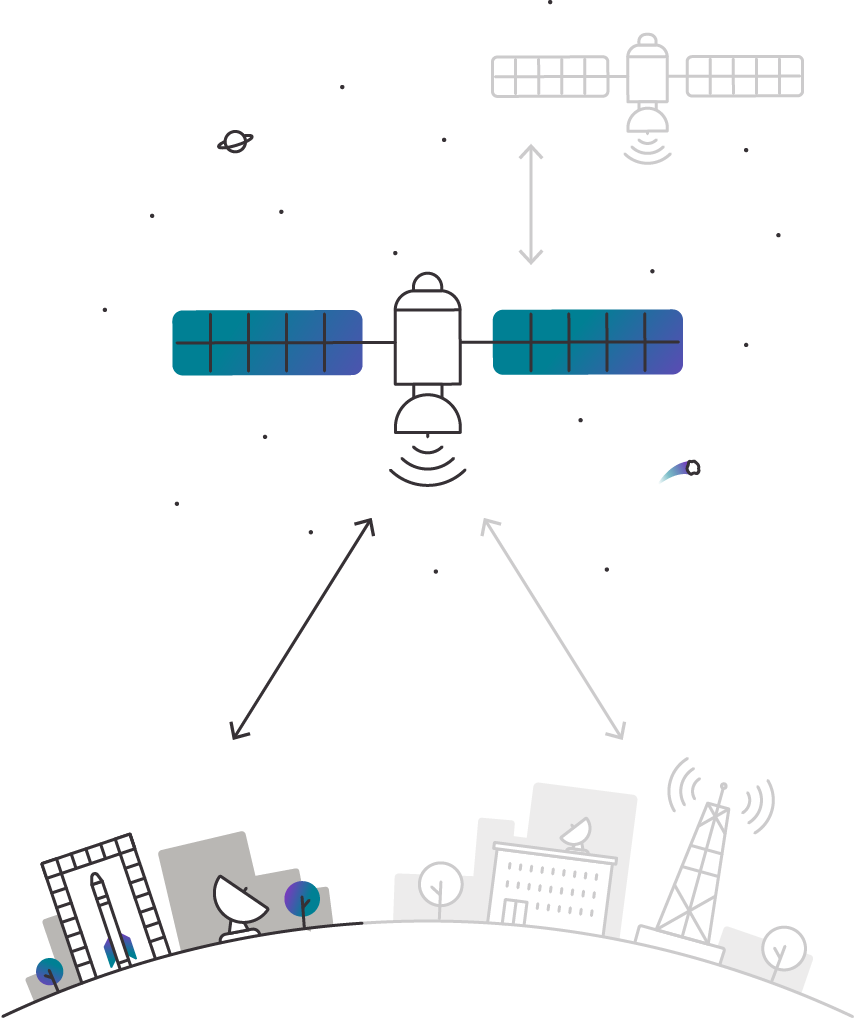
Space-based Assets
Space-based assets include communication satellites (e.g. to support 5G networks) but also other types of satellite that support systems such as positioning, navigation, and timing (PNT) (supports GPS, EU’s Galileo, Russian GLONAS) and weather satellites.
Communication satellites, and satellites in general, are comprised of a payload and a bus. The payload is mission specific and differs from satellite to satellite (i.e. the equipment necessary to perform its specific purpose such as communications). The bus provides the essential functionality (i.e. electrical power, electronics, and propulsion) enabling the mission payload. In the context of communications satellites, the mission payload is the communications package including antenna, content/data routing, and waveform management.
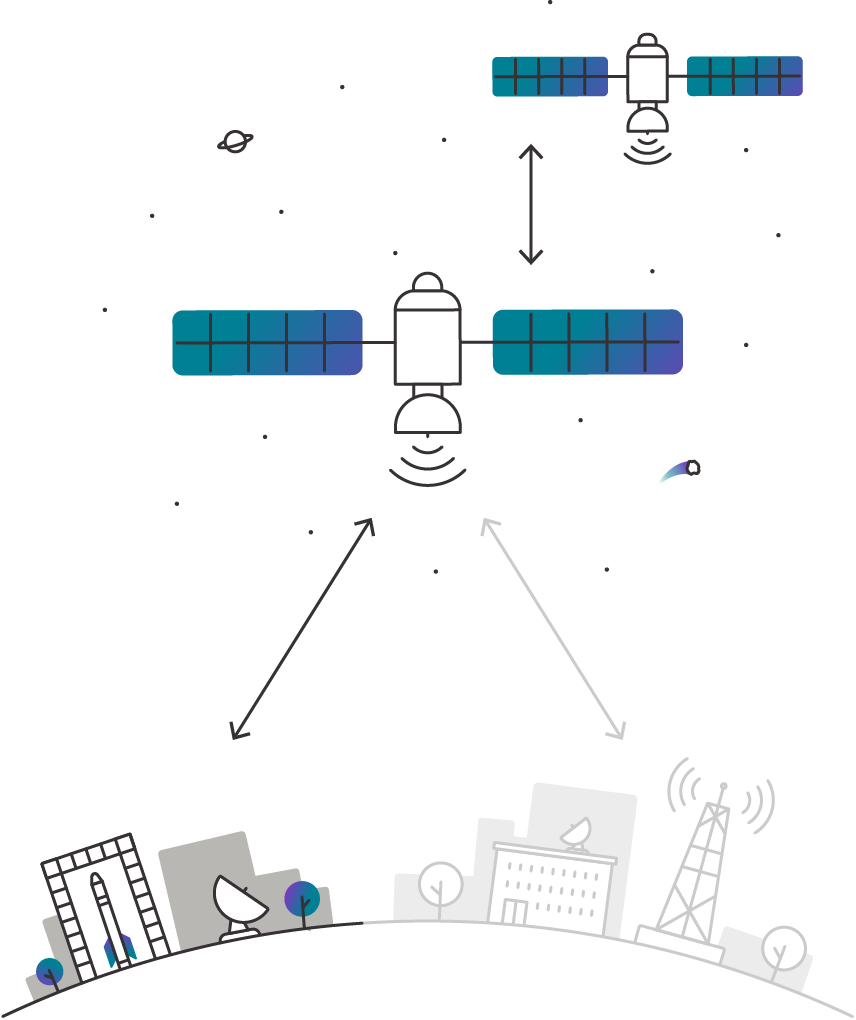
Crosslinks
In addition to uplinks and downlinks between the satellite and the ground station, some satellite constellations can also communicate with each other (crosslinks).
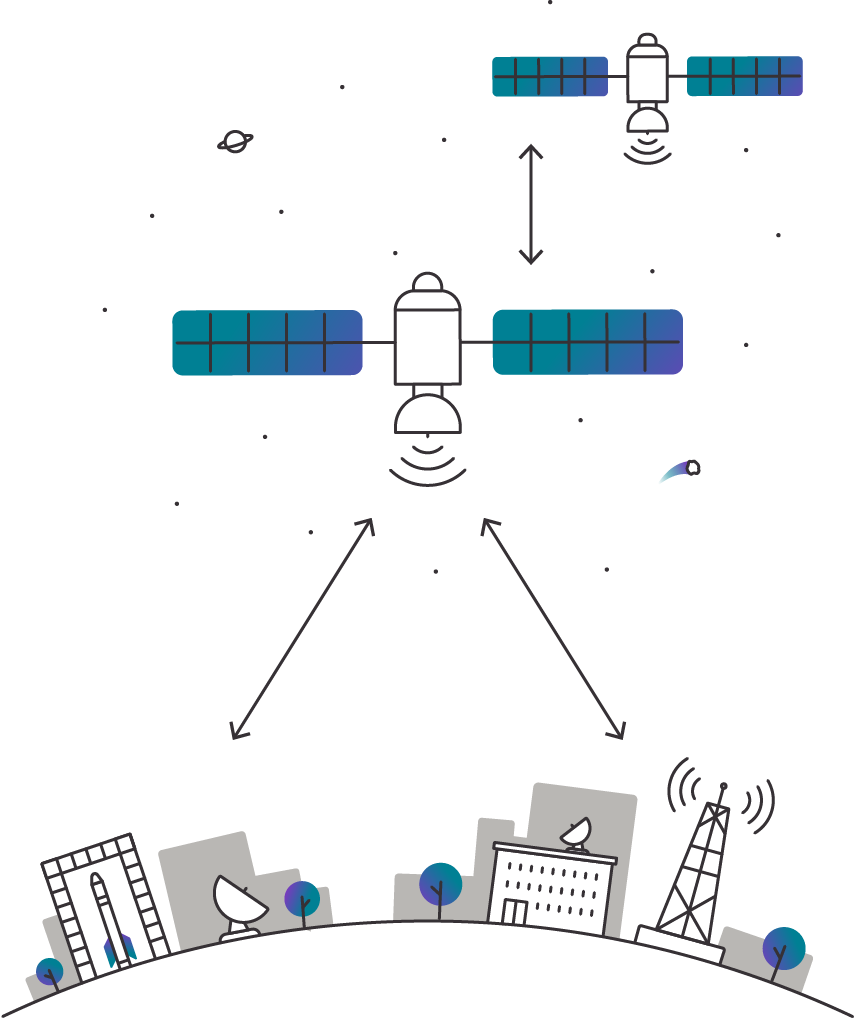
Connecting through space
Satellite systems are not an island in and unto themselves. Their utility is in their ability to connect with their users. Connection points to other networks and devices can take many forms.
In the case of serving as 5G backhaul, for example, satellite systems would connect the Radio Access Network (RAN) to the core network. Both of those connections (the RAN and core) are endpoints for the satellite system. In the case of increasing connectivity for IoT devices in an urban area, numerous IoT devices become endpoints (or connection points).
Notably, communication satellites can support commercial, government, or military purposes and often satellites can support multiple communities at the same time. For example, according to a DoD study, “commercial communications satellites were used in 45 percent of all communications between the United States and the Persian Gulf region during Desert Shield/Desert Storm.”
As an industry, satellite systems are supported by a diverse set of players across the hardware and software supply chain. As summarized in a 2002 GAO report on Critical Infrastructure Protection for commercial satellite systems:
[t]he commercial satellite industry includes manufacturers, the launch industry, service providers, and ground equipment manufacturers. Manufacturers design and build satellites, supporting systems, and ground stations. The launch industry uses launch vehicles, powered by rocket engines, to place satellites in orbit. Once commercial satellites are in orbit, they are operated by service providers, who lease available services.
Now, two decades later, the industry has evolved to also include vertically integrated providers--such as SpaceX, which currently produces everything on the operations side from their rockets to software for their satellites in house--as well as a diversity of users relying on these systems for their connectivity needs as service prices dropped with the emergence of smaller satellites and CubeSat technology, which spreads the cost of a launch across many users.
In short, the communication satellite industry ecosystem is composed of and supported by an array of vendors, operators, and users. Those vendors and operators span ground-based assets, space-based assets, links between the two, and connection points to other networks and devices (the customers). For the fifth generation of telecommunications, that ecosystem will be increasingly integrated with the territorial networks that frequently come to mind when someone says 5G - cellular towers; radio heads; fiber; servers; sensors in cars, hospitals, and factories; a plethora of mobile devices; etc. - and their supporting ecosystems.
How has the Role of Satellites in Telecommunications Networks Evolved Over Time?
Historically, satellite and terrestrial communications networks developed separately and were largely isolated from each other. Satellites have been used for backhaul in telecommunications networks for almost 30 years. However, while satellites offered significant bandwidth capacity (upwards of 1GB/s), the latency was extremely high, and required extensive physical infrastructure to move data. This meant that any high bandwidth data movement was a (i) costly, (ii) highly orchestrated, and (iii) deliberately planned activity. As a result, satellites have been a critical component for solving the “last mile” problem but, at the same time, largely limited to transmitting data to these disconnected or hard to reach locations.
Why the “Last Mile”?
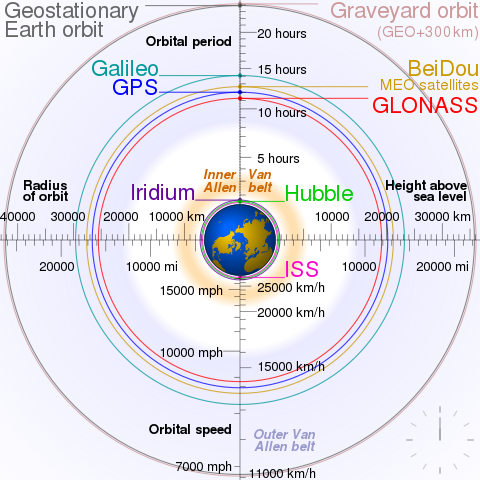
Most of those satellites sat in geosynchronous earth orbit (GSO) in earth-faced fixed inclination at roughly 36,000 kilometers above the earth. Put simply, if one is placed overhead, look up and it is always overhead and pointing down at you. The benefit of GSO orbit is that their fixed position allows for continuous coverage of a geographic area by matching the rotation of the earth. Given their altitude, a single GSO satellite also has a potentially wide field of view (or coverage area). AT&T’s DirectTV and HughesNET constellations are modern examples of a GSO constellation satellite systems. These are large traditional communications satellites (in fact, many of them have their own Wikipedia pages). Importantly, however, these GSO constellations, in general, require professional installation for the customer facing ground element and offer limited bandwidth. The fixed nature of GSOs is also one of their downsides; given their distance from the earth’s surface, GSOs tend to take longer to transmit data to and from ground-stations and devices than other closer terrestrial transmission stations. While this difference is measured in milliseconds and seconds, it creates challenges when high bandwidth, low latency connections are necessary.
What’s Changed?
In contrast to GSO satellites, non-geosynchronous orbit (NGSO) communications satellites, including low earth orbit (LEO) satellites and the particular class of emerging proliferated LEO systems like SpaceX’ Starlink constellation, are well positioned to solve more than the “last mile” problem. Unlike traditional LEO and GSO constellations, which have a small number of satellites, less than 100 in each system, the proliferated LEO (pLEO) systems, like SpaceX’s Starlink currently have closer to 1,320 and plan to have several thousand.
LEOs differ from GSOs in two important ways. First, a LEO typically sits somewhere between 160 and 2000 kilometers above the earth’s surface and these satellites fly in rapid orbits around the earth (many different orbits around the earth at various altitudes – a swarm). Put simply, with pLEOs, a specific satellite is not overhead all the time, but given their speed and numbers, the satellite constellation will be overhead constantly. Second, LEOs, given their low earth orbit, stay in orbit for a shorter period of time than GSOs. Why? Satellites sitting in LEO are subject to higher atmospheric drag. The degree to which atmospheric drag affects the lifespan of the satellite depends on where in the LEO the satellite sits (altitude - low (160 KM) to high (2000 KM)) and whether the satellite has station-keeping thrusters (can adjust its orbit over time).
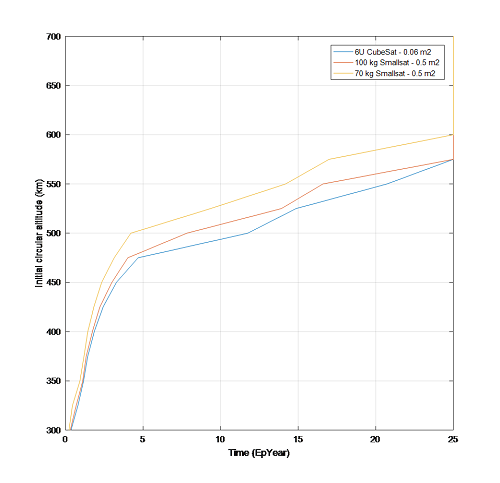
For example, depending on the satellite's altitude, atmospheric drag can lead to a fairly rapid decaying orbit or be fairly negligible in a satellite's lifespan. If a satellite is sitting at 500KM or less and dies, it deorbits in less than a decade (single digit years, typically 5 years or less). If you go above 500KM that number increases to a decade and then decades. As a result, satellites in a “low” LEO orbital plane will need some ability to make altitude adjustments, which requires operators to invest more in their systems. As a consequence, pLEO systems are considerably smaller and built to be disposable (often with a design life of about five years).
A useful byproduct of a decaying orbit is that it makes it easier to get rid of space junk (satellites that are obsolete or no longer in use). This proves to be a fairly important byproduct when we move from GSOs to pLEOs (swarms rather than smaller satellite constellations). For example, SpaceX deploys (or injects) their satellites in low LEO orbit (around 280KM) before maneuvering them to a higher LEO orbit (about 550KM). This allows them to use atmospheric drag to address any issues that become apparent immediately after deployment (at that altitude without propulsion, the satellite is gone in a few days) and they can deorbit satellites if issues arise later or as a satellite nears the end of its lifecycle (SpaceX expects their satellites to last anywhere between 5-7 years) so that drag works in their favor.
Why Didn’t We Launch Swarms of LEOs Earlier?
The physics of pLEO is favorable for launching many small, relatively disposable systems. Yet, until recently, the necessary combination of launch technology and the electronics, both on the ground and on the satellite, was not affordable or available.
Another significant factor impacting the deployment of LEO systems is the ability of the satellite to “see” the earth. GSO satellites, in a relatively fixed position around the earth, can see roughly a third of the earth and have historically been designed to be in near-constant use albeit with higher latency. LEO satellites, because of their size, much lower altitude, and constant orbit around the earth, see the earth through a soda straw with a much smaller area of regard on the earth and are designed to broadcast for 15-20 minutes per orbit but with much lower latency, hence the need for swarms. LEOs are smaller than their GSO counterparts, and that smaller size coupled with the reality that launching a satellite into a lower orbit is less costly than launching that same satellite into higher orbit makes them cheaper to lift into space. But the cost savings do not end there. Private operators, namely Blue Origin’s New Shephard and SpaceX’s Falcon 9, have also been able to drive significant launch cost reductions by reusing launch vehicles, which reduces one of the most expensive elements of putting anything into orbit.
One important effect of lowering launch costs is that it reduces the pressure on operators to launch as sparingly as possible. Companies such as SpaceX have radically transformed the launch space. By way of comparison, AT&T operates a GEO constellation of 14 satellites, each requiring a dedicated launch because each satellite weighs on average 5,600 kilograms with a design life of 15 years. Contrast this with SpaceX’s Starlink service, which launches 60 satellites, per launch into LEO because each satellite weighs 227 kilograms and is designed for a shorter lifespan. As of March 2021, “SpaceX now has 1,200 Starlink satellites in orbit, having launched 310 of them [in 2021] alone. Five of SpaceX’s seven Falcon 9 missions in 2021 have been dedicated for Starlink, with the other two launching Transporter-1 and the Turksat 5A geostationary communications satellite.” Keep in mind, that by the time you read this sentence, the number of Starlink satellites in orbit will be even greater.
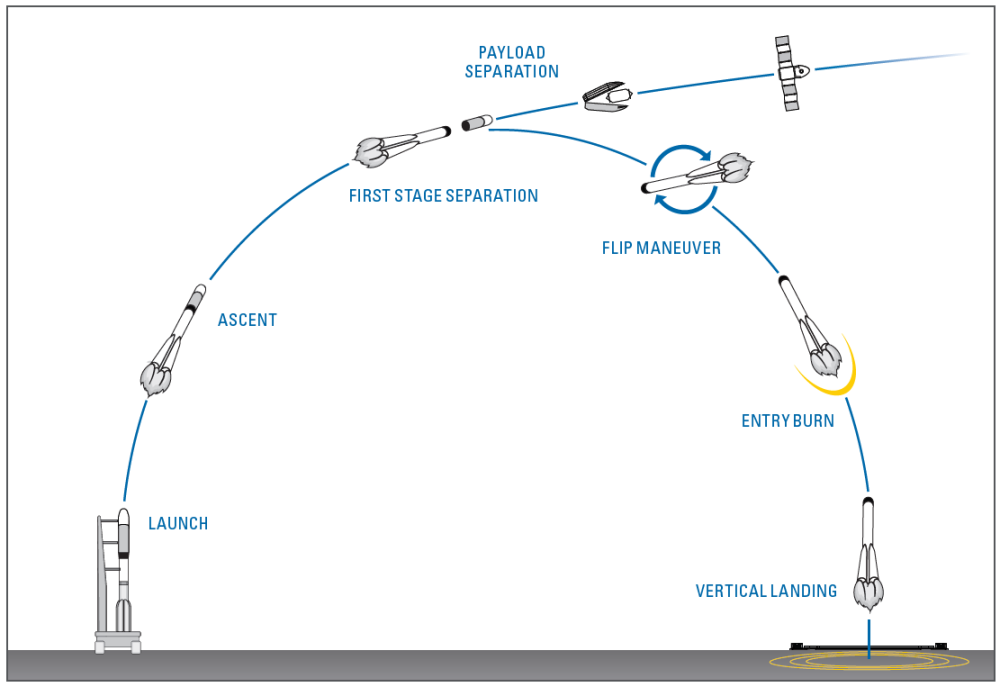
Notably, not all satellite communication constellation companies have their own launch capabilities. While Amazon’s Kuiper constellations are still being designed, the company gained U.S. Federal Communications Commission (FCC) approval last year (2020) to operate a constellation of approximately 3,200 internet satellites in LEO by July 30, 2029 (Amazon must launch 50% of its constellation by July 30, 2026 to maintain its authorization). To inject those satellites into orbit, Amazon recently selected United Launch Alliance’s (ULA) Atlas V rockets for at least nine of its launches.
Advancements are not just limited to the launch space, however. Current and legacy advances in electric propulsion systems and microelectronics, such as the development and availability of cost-effective active electronic phased arrays, field programmable gate arrays, and application specific circuits (a type of semiconductor chip or integrated circuit), have accelerated the design and deployment cycles as well as the capabilities of satellites.
Why are pLEOs an Advancement?
A swarming constellation of pLEOs would provide uninterrupted, real-time, ubiquitous coverage of a region. This in and of itself is a significant shift in how we think about satellite communications. Unlike legacy systems where it was costly and complicated to move data around the earth using GSOs for the “last mile” while still relying heavily on terrestrial fiber, pLEO creates the opportunity to move data around the earth without using terrestrial fiber.
The advancement pLEOs represent is also a story of the business models satellite companies can now pursue. Companies can now approach satellite systems as a service to be sold on to customers rather than a bespoke, sustained business investment that a customer needs to make. For example, Amazon Web Services (AWS) now offers a “fully managed service that lets you control satellite communications, process data, and scale your operations without having to worry about building or managing your own ground station infrastructure.” In other words, AWS has built a business model around “Ground Station as a Service” that you purchase and then seamlessly integrate into your business operations rather than ground stations as a bespoke investment that you need to invest in and acquire specific equipment to access, tailor to your specific use-case, and then maintain those assets over time. Flexible payloads, such as those developed by Airbus, are another example: offering operators the capability to reprogram satellites’ missions (e.g. reconfigure its frequencies, coverages and/or power allocation) after the spacecraft is in orbit. In short, satellite systems of the past were mission specific and high resource investments. The satellite systems of today, which are currently disrupting that market, strive to be plug-and-play across a wide diversity of telecommunications needs.
What’s the End Result?
With swarms of LEOs and satellites as a service on the horizon, 5G networks have a viable alternative to fiber for real-time data backhaul. This provides utility for expanding coverage in areas where laying fiber is not economically viable (remote) or feasible (a ship or an airplane). It also provides redundancies and alternatives to backhaul in more urban areas where fiber has already been laid and has the potential to increase connectivity to accommodate an ever-increasing number of connected devices and data traffic (the unique demand an explosion in IoT devices will bring). In short, with the evolution of satellite systems and the requirements of the future 5G proponents promise, 5G will need to rely on an integrated telecommunications system with both terrestrial and space-based components working in tandem.
What Purpose Could Satellites Serve in 5G Networks?
In order to deliver on the full promise of 5G networks (near ubiquitous, instantaneous coverage for a massive number of connected devices), satellites will need to play a far more central role within telecommunications networks going forward with both terrestrial and space-based components working in tandem for a wider diversity of functions. Given the evolution of the satellite industry, both in terms of business models and technology, that greater role is now, for the first time, possible.
Notably, the specific and diverse roles satellites will play in the future is still an open question and the answer depends as much on industry and business decisions as it does on technological and economic feasibility. However, in 5G networks, satellites could serve three potential functions: providing additional backhaul, creating redundancies, and providing remote and rural areas with greater connectivity. In each of these cases, there is a diversity of business models that could potentially emerge from direct to device connections to connections between the end-user and the core network.
Backhaul
Historically, backhaul (moving data between the radio access network (RAN) and the core network) primarily occurred over fiber or wireless point-to-point. However, increasing demands on telecommunications networks have increasingly incentivized “mobile network operators around the globe to evaluate different backhaul technologies to meet the rapidly increasing demand for their 4G/LTE network deployments.” The need for a greater diversity of backhaul options will only increase as 5G deployments progress. Why? As the number of small cells (low-power, short-range base stations (wireless transmission systems) covering limited geographic areas) in the radio access network (RAN) increases, so too does the demands on backhaul between the RAN and the core network. Now, with swarms of LEOs on the horizon, 5G networks have a viable alternative for real-time data backhaul. Given the demands on 5G networks and the evolution of satellite systems, it is possible for satellites to complement existing backhaul mechanisms to meet the growing demand.
Redundancy
In addition to increased demands on backhaul, with the move toward pLEOs, satellites now have the potential to provide overlay networks duplicating segments of the terrestrial networks. This overlay network could replace or augment existing terrestrial networks if those networks experience reduced functionality due to man-made (physical or cyberattacks but also simply mistakes/accidents) and natural disasters. While their utility would be limited, they could potentially prioritize critical services and buy operators time to restore access to terrestrial networks. In short, given that 5G networks will be essential for the daily functioning of not only our economy but society, government, and military, they represent a potential single and catastrophic point of failure. Satellite systems overlaying aspects of terrestrial systems deemed strategically important or essential for emergency operations in the event of a catastrophe, can provide those systems with additional resiliency through redundancy.
Remote and Rural Connectivity
Historically, GSOs have been critical for solving the “last mile” problem. With the proliferation of swarms of LEOs, that role could increase both in terms of scale (number of connections) and scope (where those connections can be made). 5G networks will bring with them an exponentially growing number of connected devices, including mobile phones but also a vast array of IoT devices including billions of sensors. Think rural hospitals carrying out remote surgeries; cars traversing interstates, planes in flight, and ships out at sea; and agriculture fields full of sensors. While their utility in dense urban areas is more limited (satellites require line of sight for a direct connection to a device), the opportunity now for Massive Machine Type Communications (MMTC) and the fact that many of these devices will be scattered over wide geographic areas increases demands on data collection and distribution across 5G networks. Here too, satellites, integrated into terrestrial telecommunications networks through new network architectures, can provide an important solution by leveraging the wide satellite coverage enabled by pLEOs. In this regard, one of the biggest benefits is that LEOs, unlike GSOs, can now deliver a real interactive experience, a degree of connectivity often lacking in rural and remote areas to date. With the addition of more fully integrated satellite systems into 5G networks, connectivity can be expanded to remote areas where laying fiber is not economically viable (communities across the U.S. and as well as around the world) or feasible (an oil rig off the coast, a ship traversing the ocean, or an airplane flying overhead).
Conclusion
In conclusion, the promise of satellite systems for 5G networks rests on their ability to help us increase the scale and scope of access to these networks; meet increasing demands on these networks, especially in rural and remote areas; and incorporate redundancy in critical segments of these networks. Evolutions in underpinning technology and the diversity of business models companies can now pursue makes this integration not only possible but feasible. Though this outcome is far from certain and would require investment in and a willingness by both the satellite and 5G communities, this goal has increasingly become a sustained focus of the technical standards bodies, researchers, and industry in an effort to make the 5G promise a local and global reality.
The views expressed are those of the authors and do not reflect the official guidance or position of the United States Government, the Department of Defense or of the United States Air Force.
Authors
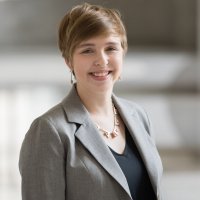
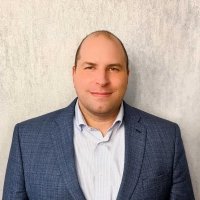
Science and Technology Innovation Program
The Science and Technology Innovation Program (STIP) serves as the bridge between technologists, policymakers, industry, and global stakeholders. Read more
Explore More
Browse Insights & Analysis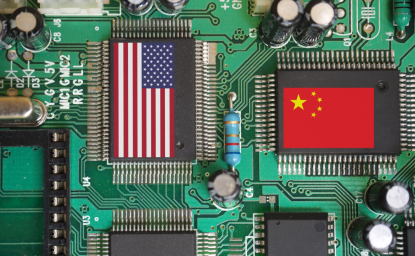
360° View of How Southeast Asia Can Attract More FDI in Chips and AI
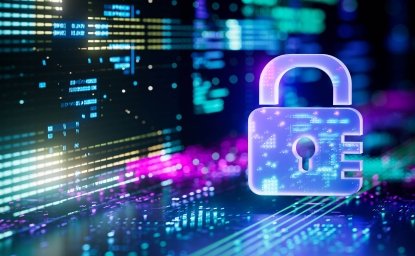